Newly Designed Material Could Make Sound Travel Backward
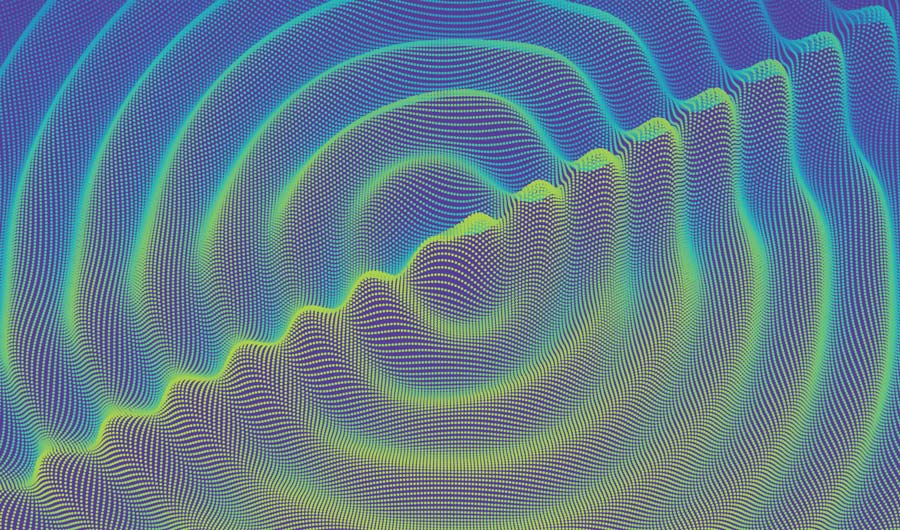
(Inside Science) -- Think of a moonwalk. The dancer moves backward even though they look like they are walking forward. In the quantum world, waves can do a similar thing: Backward waves move in one direction while their energy moves the other way.
A team of scientists has proposed a new type of material that could create these moonwalking waves when transmitting sound. They published their design in the journal Nature Communications this June, and they plan to announce the successful creation of the material in a new paper coming soon.
The material belongs in a category called metamaterials, which is a catch-all term for any artificial substance that has a property not seen in nature. People’s interest in these surprising creations started over a century ago, said Andrea Alù, a metamaterials researcher at The City College of New York, who was not involved in the new research. That interest has spiked in the last couple of decades, he said, because scientists have become capable of fabricating materials on a nanometer scale with high precision. And the excitement for metamaterials has expanded beyond the scientific community as the research started producing real versions of invisibility cloaks.
The team behind the new addition to the metamaterial portfolio was inspired by superfluid helium-4: a liquid form of helium with no viscosity that can only be observed at an extremely low temperature of around minus 450 degrees Fahrenheit. In addition to having the ability to climb up a wall, superfluid helium-4 also exhibits the acoustic backward-wave phenomenon.
However, the researchers’ goal wasn’t to copy superfluid helium-4, said Martin Wegener, a metamaterials researcher at Karlsruhe Institute of Technology in Germany and the senior author of the paper. Instead, he and his team wanted to design a new material from scratch. The team first imagined a simple one-dimensional model with dots and springs, standing in for atoms and bonds. In the model, neighboring dots were connected to each other via springs, forming a row. Since the scientists had deduced from their previous research that the key to backward-wave behavior lies in faraway interactions, the team also added stiffer springs that would connect third-nearest dots. When the team ran mathematical simulations using their model, it exhibited the behavior that the team was looking for: backward waves.
To fabricate a real-life metamaterial, however, the team would have to transform their one-dimensional model into a 3D design -- a difficult task, Wegener said. The researchers substituted dots with cubes and springs with bars, and designed a lattice structure where the neighboring cubes are connected vertically, while the third-nearest neighboring cubes are connected via diagonal and horizontal bars. According to the team’s calculations, if the design were to be 3D-printed with precision, the resulting metamaterial would show the ripple of the sound wave moving in the opposite direction of the energy flow.
Alù said Wegener’s and his team’s design exhibited a counterintuitive geometry that scientists wouldn’t immediately think of experimenting with. Typically, when atoms or molecules interact with each other in a material that has a repeating pattern, as in Wegener’s new design, they interact mostly through their nearest neighbors, Alù said.
The jury is still out on how the new metamaterial would perform in real life, or what purpose the backward-wave phenomenon of sound waves would serve. "We and many other people in the field of metamaterials are still playing with the possibilities," said Wegener. "We're trying to find out what is it that we can do beyond what ordinary materials have to offer."
Wegener said he and his team successfully brought the design to life, though he couldn’t share the details because the results will be announced in their next paper.
Though this metamaterial was developed without a specific application in mind, metamaterial research is typically driven by unmet needs that traditional materials cannot satisfy, said Alù. The science of making materials with seemingly impossible properties may also lead to discoveries and applications that have yet to be envisioned, he said. "It's a beautiful field because … it gives you this opportunity to make an impact on technology."